Related Projects
About the Microfluidics Lab
The presentation of the Microfluidics Lab is bipartite: On the one hand, the microfluidic cells have to be prepared in an extensive workflow, including optical and soft lithography. On the other hand, the pore- respectively REV-scale imaging of the processes taking place inside the manufactured microfluidic cells can be performed with different (both in-house-built and commercial) microscopes.
Production of microfluidic cells
The first step to produce microfluidic cells consists of optical lithography: In the cleanroom from the Department of Physics, silicon wafers are spin coated with photoresist, exposed to UV illumination and finally developed. The features of the photoresist profile are measured in order to assure of the quality before the wafer is ready to be used as a mold for the subsequent soft lithography step.
Next, in the soft lithography part, the Poly-Di-Methyl-Siloxane (PDMS) elastomer base is mixed with the corresponding curing agent. After inital degassing, two equal portions of the mixture are poured into two petri dishes, where one of them contains the silicon wafer and the other is left blank. Omitting intermediate steps (further degassing in vacuum chamber, curing at 70°C in oven), the two hardened PDMS slabs (after removal of the silicon wafer) are bonded together to constitute the microfluidic cell.
With this workflow, the manufactured cells exhibit features at a microscale resolution of below 2 µm, with minimum feature size in the order of 20 µm, depth of the porous structure of up to 120 µm and overall size in the order of a few centimeters.
Imaging techniques and microscopes
To characterize the physical actions taking place on the pore-/REV-scale inside the microfluidic cells, the PML is equipped with a number of microscope compositions.
- A three-camera, in-house-built microscope can record images from elongated samples with a width-to-length ratio of 1:3. The series of three identical Basler acA2440-20gm cameras generates images at frame rates ranging from 0.044 to 23 fps and a resolution from 0.5 to 20 µm per sensor pixel.
- Another in-house-built microscope including only one camera with an identical resolution as beforehand is present in the PML. In this case, the frame rate can be defined between 0.044 to 700 fps. Therefore, depending on the acquisition rate, the cameras Allied Vision Prosilica GC2450, Basler a2A1920-160umBAS and Optronis Cyclone-5-700-M are available.
All components (cameras, lenses, beam splitters etc.) of the above introduced setups are interchangeable. Both microscopes can operate in transmitted (for transparent microfluidic devices) or reflected (for partially transparent samples) mode. There are, of course, further possible variations (i.e. illumination source) and boundary conditions to guarantee a smooth and reliable measuring operation. An additional tabletop microscope, this time using coaxial illumination, is mostly used to perform static experiments like contact angle measurement or quality control for the microfluidic devices.
- This microscope can host numerous cameras, for instance IDS UI-3070CP-C-HQ Rev. 2, IDS UI-5250SE-M-GL Rev. 2 or Basler acA5472-17um. The degrees of freedom regarding the modularity are limited compared to the open microscopes, but apart from that, the higher optical resolution is the key feature to highlight.
Finally, the PML offers a commercial, but still somewhat modular µ-PIV system. The provided modules are the following:
- A Zeiss SteREO Discovery.V12 modular stereo microscope represents the basis with the possibility of remote control from a computer. The fluorescence module from LaVision, namely the Bernoulli Nd:YAG double-pulsed laser, together with the LaVision Imager sCMOS permit µ-PIV imaging with maximally 25 (double-valued) fps.
Altogether, the µ-PIV system reaches a spatial resolution of 1 µm per sensor pixel and a temporal resolution of around 120 ns (meaning that the velocity profile of some flow scenario can be quantified for Reynolds numbers at at least Re > 2000).
Experiments
Enzymatically Induced Calcite Precipation in a microfluidic cell
Left: Segmented images of the whole cell (bottom) and an enlarged segment of it (top).
Right: Corresponding 2d porosity reduction (top) and pressure measurements (bottom).
PIV for flow of a fluid in a fracture of 1mm aperture (including surrounding porous medium)
Left: Aquired raw images, converted to a video at 25 fps.
Right: Colored, derived velocity field from the measured raw images (blue = low / red = high velocity magnitude).
Drainage event in a microfluidic device
The non-wetting phase is water dyed with ink, and the wetting phase is Fluorinert FC-43. The water has an elevated dynamic viscosity of µ = 50 Pa·s through the addition of Glycerol, creating a viscosity ratio of 10. The capillary number is Ca = 10-2.
Simultaneous thermal and optical imaging of a displacement experiment
The phases involved are Fluorinert FC-43 (as the wetting phase) and water dyed with ink (as the non-wetting one). Warm water is introduced into the flow network by suction to minimize temperature losses. As PDMS tends to absorb wavelengths which correspond to the emitted heat, the microfluidic device comprises a PDMS slab with the features of the flow network embedded and a PDMS spin coated (10 µm thick) Kapton film to enable thermal visualization. Both drainage and imbibition take place, induced by reversing the flow direction.
Publications
- Weinhardt, F., Class, H., Vahid Dastjerdi, S., Karadimitriou, N., Lee, D., Steeb, H. (2021). Optical Microscopy and pressure measurements of Enzymatically Induced Calcite Precipation (EICP) in a microfluidic cell. DaRUS, V1. doi:10.18419/darus-818
- Vahid Dastjerdi, S., Steeb, H., Ruf, M., Lee, D., Weinhardt, F., Karadimitriou, N., Class, H. (2021). micro-XRCT dataset of Enzymatically Induced Calcite Precipation (EICP) in a microfluidic cell. DaRUS, V1. doi:10.18419/darus-866
- Gao, H., Tatomir, A. B., Karadimitriou, N., Steeb, H., Sauter, M. (2021). A two‐phase, pore‐scale reactive transport model for the kinetic interface‐sensitive tracer. Water Resources Research, 57. doi:10.1029/2020WR028572
- Wagner, A., Eggenweiler, E., Weinhardt, F., Trivedi, Z., Krach, D., Lohrmann, C., Jain, K., Karadimitriou, N., Bringedal, C., Voland, P., Holm, C., Class, H., Steeb, H. (2021). Permeability estimation of regular porous structures: a benchmark for comparison of methods. Transport in Porous Media, 138, 1-23. doi:10.1007/s11242-021-01586-2
- Weinhardt, F., Class, H., Vahid Dastjerdi, S., Karadimitriou, N., Lee, D., Steeb, H. (2021). Experimental Methods and Imaging for Enzymatically Induced Calcite Precipitation in a Microfluidic Cell. Water Resources Research, 57. doi:10.1029/2020WR029361
- von Wolff, L., Weinhardt, F., Class, H., Hommel, J., Rohde, C. (2021). Investigation of Crystal Growth in Enzymatically Induced Calcite Precipitation by Micro‐Fluidic Experimental Methods and Comparison with Mathematical Modeling. Transport in Porous Media,137, 327-343. doi:10.1007/s11242-021-01560-y
- Yiotis, A., Karadimitriou, N., Zarikos, I., Steeb, H. (2021). Pore‐scale effects
during the transition from capillary‐ to viscosity‐dominated flow dynamics within microfluidic
porous‐like domains. Scientific Reports, 11. doi:10.1038/s41598-021-83065-8 - Konangi, S., Palakurthi, N. K., Karadimitriou, N., Comer, K., Ghia, U. (2021). Comparison of pore-scale capillary pressure to macroscale capillary pressure using direct numerical simulations of drainage under dynamic and quasi-static conditions. Advances in Water Resources, 147. doi:10.1016/j.advwatres.2020.103792
- Hasan, S., Niasar, V., Karadimitriou, N., Godinho, J. R. A., Vo, N. T., An, S.,
Rabbani, A., Steeb, H. (2020). Direct characterization of solute transport in unsaturated porous media using fast X-ray synchrotron microtomography. Proceedings of the National Academy of Sciences, 117, 23443-23449. doi:10.1073/pnas.2011716117 - Karadimitriou, N., Mahani, H., Steeb, H., Niasar, V. (2019). Nonmonotonic Effects of Salinity on Wettability Alteration and Two-Phase Flow Dynamics in PDMS Micromodels. Water Resources Research, 55, 9826-9837. doi:10.1029/2018WR024252
- Yin, X., Zarikos, I., Karadimitriou, N., Raoof, A., Hassanizadeh, S. M. (2019). Direct simulations of two-phase flow experiments of different geometry complexities using Volume-of-Fluid (VOF) method. Chemical Engineering Science, 195, 820-827. doi:10.1016/j.ces.2018.10.029
- Hasan, S., Joekar‐Niasar, V., Karadimitriou, N., Sahimi, M. (2019). Saturation Dependence of Non-Fickian Transport in Porous Media. Water Resources Research, 55, 1153-1166. doi:10.1029/2018WR023554
For further information please contact
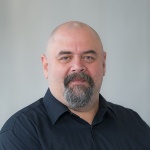
Nikolaos Karadimitriou
Dr.Research associate
[Photo: Max Kovalenko]

Felix Weinhardt
Dr.-Ing.Postdoc
[Photo: Felix Weinhardt]